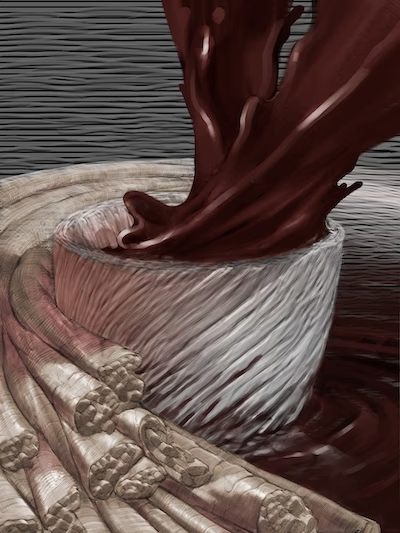
The custom-designed three-dimensional printed model showcases a detailed representation of a human coronary heart ventricle, utilizing cutting-edge technology and innovative materials by incorporating fibers into the printing ink.
In recent years, breakthroughs in three-dimensional printing technology have paved the way for bioengineers to create complex biological structures, including functional heart tissue models, as well as architectural prototypes for building construction. Their objectives encompass developing higher platforms for identifying novel therapeutics for coronary heart disease, the leading cause of death in the US, responsible for approximately one in five fatalities nationwide, and leveraging 3D-printed cardiac tissue models to predict which treatments will be most effective on an individual patient basis. Researchers aim to develop implantable biomaterials capable of inducing the repair or regeneration of damaged or diseased cardiac tissue within an individual’s own heart.
Researchers from SEAS and the Wyss Institute for Biologically Inspired Engineering at Harvard University have reported the development of a novel hydrogel ink infused with gelatin fibres, enabling the 3D printing of a functional heart ventricle that simulates beating like a human heart. Researchers discovered that the fiber-infused gel (FIG) ink enables cardiac muscle cells printed in the shape of a ventricle to synchronize their contractions, mimicking the coordinated beating of a human heart chamber?
“Researchers are striving to replicate organ functionality in a controlled environment to ensure the integrity of drug testing and predict potential outcomes in a scientific context,” remarks Suji Choi, research associate at SEAS and lead author on the study. Although current 3D printing techniques have not been sufficient to achieve physiologically relevant alignment of cardiomyocytes, the cells responsible for transmitting electrical signals in a coordinated manner to contract cardiac muscle.
“We embarked on a mission to address the limitations in 3D printing of organic tissues.”
– Kevin “Equipment” Parker
The game-changing innovation emerges from the integration of fibers into a printable ink. According to Choi, FIG ink successfully flows through the printing nozzle, retaining its three-dimensional shape once the construction is printed. “Given the unique properties of this material, I found that it is possible to create complex 3D structures, including ventricles, without requiring additional support materials or scaffolding.”
Choi leveraged a novel technology developed in his laboratory by a team led by Dr. That utilizes a strategy akin to spinning cotton thread to manufacture microfiber supplies. Postdoctoral researcher and Wyss Lumineer Luke MacQueen, a key contributor to the study, originally suggested the innovative idea of incorporating fibers produced through the rotary jet spinning process into ink, allowing for their subsequent 3D printing application. As a Wyss Affiliate School member and the Tarr Household Professor of Bioengineering and Applied Physics at the Harvard John A. Paulson School of Engineering and Applied Sciences (SEAS), Parker plays a significant role in advancing interdisciplinary research and education.
“When Luke conceived his concept, the vision was to expand the range of printable spatial scales using 3D printing technology by pushing the lower bounds downwards, effectively reaching the nanoscale.” “The key advantage of using rotary jet spinning to manufacture fibers over electrospinning, a more conventional method for creating ultra-thin fibers, is that it allows us to utilize proteins that might otherwise be damaged or degraded by the electrical fields inherent in the electrospinning process.”
Choi successfully spun gelatin fibers using a rotary jet, thereby creating a fabric that mimicked the appearance of cotton in its texture and visual characteristics. Using sonification, she converted the sheet into fibers approximately 80-100 micrometers in length and 5-10 micrometers in diameter. She then dispersed these fibers directly into a hydrogel ink.
Our cutting-edge approach ensures seamless production of custom-length fibers with precise control over shape and form.
– Suji Choi
What proved particularly challenging was optimizing the delicate balance between fibers and hydrogel within the ink, ensuring precise alignment of fibers and maintaining the overall structural integrity of the three-dimensional printed construct.
As researchers employed FIG ink to print 2D and 3D structures, the cardiomyocytes aligned themselves in synchrony with the fibre pathways embedded within the ink. By governing the printing pathway, Choi could thereby regulate the alignment of central muscle cells.
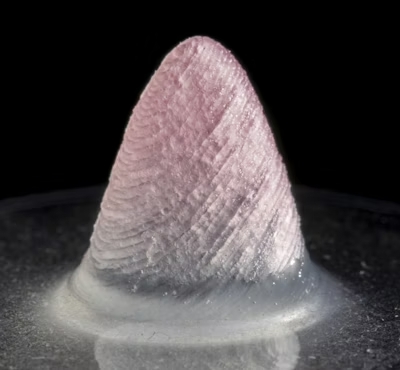
The tissue-engineered 3D ventricle mannequin.
As she employed electrical stimulation to structures composed of FIG-ink printed buildings, she found that it provoked a synchronized contraction pattern aligning with the fibre paths. As he observed the ventricle-shaped structure, Choi’s excitement grew: “It was incredibly exhilarating to witness the chamber mimicking the natural pumping mechanism of actual heart ventricles.”
As she pushed the boundaries of printing techniques and ink chemistry, she found that introducing subtle variations in design and composition could yield remarkably robust fibers within intricately patterned cavities.
“While our ventricle model is a simplification and downsizing of the actual coronary heart, she notes.” Researchers are now focused on developing more realistic cardiac tissue constructs with robust muscular layers that can effectively propel fluid with increased pressure. While not as biomechanically complex as native cardiac tissue, the novel 3D-printed ventricles have been shown to potentially pump 5-20 times more fluid volume than previous iterations of printed heart chambers.
The staff predicts that this innovative approach will also be employed in the development of coronary heart valves, dual-chambered miniature hearts, and other applications.
Parker notes that FIGs represent a tool they’ve created specifically for additive manufacturing. We’ve developed diverse approaches to enhance tissue engineering capabilities, enabling us to pursue innovative regenerative therapies with greater precision and effectiveness. Our goal is to transcend tool reliance – we adopt an unbiased stance in pursuit of innovative biological construction methods.
Authors such as Keel Yong Lee and Sean L further solidify this notion. Kim, Huibin Chang, John F. Zimmerman, Qianru Jin, Michael M. Peters, Herdeline Ann M.
Ardoña, X. J., Heiler, A.-C., Gabardi, R., Richardson, C., & T. W. Pu, and Andreas Bausch.
This work was supported by the SEAS, the National Science Foundation via the Harvard University Materials Research Science and Engineering Center (DMR-1420570, DMR-2011754), the National Institutes of Health and National Center for Advancing Translational Sciences (UH3HL141798, 225 UG3TR003279); the Harvard University Center for Nanoscale Systems, a member of the National Nanotechnology Coordinated Infrastructure Network (NNCI) supported by the National Science Foundation (ECCS-2025158, S10OD023519), and the American Chemical Society’s Irving S. Sigal Postdoctoral Fellowships.
Harnessing the innovative concepts inherent in Nature’s design principles to engineer bioinspired materials and systems that can revolutionize pharmaceuticals and foster a more environmentally conscious future.
Wyss Institute
Harnessing the ingenious principles of Nature, scientists are creating innovative biomimetic materials and systems that can revolutionize pharmaceuticals and pave the way for a more eco-friendly future?